➔ Index of ⦁ Introduction to Electronic Devices ⦁
Electronic Devices - Part II: Atomic models
Before treating electronic device theory, some important historical information is provided
Model of the atom
Without an accurate model of the matter, it wouldn't have been possible to develop any electronic device; electronics relies on all those theories developed at the beginning of the XX century, related to quantum mechanics. A deeper knowledge of the matter, a more accurate model of its states and its interaction with energy were gained and with this new branch of physics a lot of connections between chemistry, thermodynamics, classical mechanics, optics, electricity, electromagnetism and so on were found: scientists found out that every physical phenomenon could be explained using quantum mechanics!
Before understanding the interaction between atoms, molecules and 'light packets' (photons), an accurate atomic model had to be developed: many of them were created and proposed by different scientists but the majority of them didn't fully work: each model tried to explain a particular aspect of the atom and observed phenomena but failed to describe other ones.
Greek physicists
The first idea that the whole matter is composed of very small corpuscles dates back to 300B.C. when atomist philosophists described the atom as an indivisible entity, eternal and indestructible (the word itself, a-tomos, means uncuttable). They thought that every object and living being was made of a combination of four atom types, air, water, earth and fire, and each of them had its own shape and properties. A very good intuition for the time!
Dalton
The Dalton atom model, proposed by the English chemist John Dalton in the early 19th century, laid the foundation for modern atomic theory. Dalton's model postulated that atoms are indivisible and indestructible particles that constitute all matter. According to Dalton, atoms of different elements have different masses and properties, and chemical reactions involve the rearrangement of atoms to form new substances. Dalton's model also introduced the concept of atomic weight, with each element having a unique atomic mass based on the relative weights of its constituent atoms. While Dalton's model provided a significant advancement in understanding the nature of matter, it was later refined with the discovery of subatomic particles such as protons, neutrons, and electrons, leading to the development of more complex atomic models. Nonetheless, Dalton's contributions paved the way for further advancements in chemistry and physics, establishing the fundamental principles of modern atomic theory.
Avogadro
The Avogadro atom and matter model, proposed by the Italian scientist Amedeo Avogadro in the early 19th century, revolutionized our understanding of matter nature and paved the way for the development of modern chemistry. Avogadro's key insight was that equal volumes of gases, under the same conditions of temperature and pressure, contain the same number of molecules. This principle, known as Avogadro's law, laid the foundation for the concept of mole, a unit of measurement representing a specific number of particles (atoms, molecules, ions, etc.) in a substance. Avogadro's model helped to reconcile the differences between atomic and molecular weights, allowing chemists to accurately determine the relative masses of different elements and compounds. Furthermore, Avogadro's contributions contributed to the development of the kinetic theory of gases and provided crucial support for the atomic theory proposed by John Dalton. Overall, Avogadro's atom and matter model played a pivotal role in advancing our understanding of the microscopic properties of matter and its behaviour, shaping the field of chemistry as we know it today.
Thomson
The Thomson atomic model, proposed by the British physicist J.J. Thomson in the late 19th century, revolutionized our understanding of the structure of atoms. Thomson's model, also known as the "plum pudding" model, suggested that atoms consist of a positively charged sphere with negatively charged electrons, akin to raisins in a pudding. This model was based on Thomson's discovery of the electron through his experiments with cathode rays. Scientific data supporting the Thomson atomic model came from various sources, including Thomson's experiments and observations. For instance, Thomson's measurements of the charge-to-mass ratio of electrons provided critical evidence for the existence of subatomic particles within the atom. Additionally, observations of cathode ray deflection in electric and magnetic fields supported the idea of negatively charged electrons embedded in a positively charged sphere. While the Thomson atomic model was later superseded by more refined models such as the Rutherford model, it represented a significant milestone in the development of atomic theory and contributed to our understanding of the structure of matter at the atomic level.
Rutherford
The Rutherford atomic model, proposed by the New Zealand physicist Ernest Rutherford in the early 20th century, revolutionized our understanding of atomic structure. Rutherford's model suggested that atoms consist of a dense, positively charged nucleus surrounded by orbiting electrons, analogous to the solar system. This model was based on Rutherford's famous gold foil experiment, conducted with his collaborators Hans Geiger and Ernest Marsden. In this experiment, alpha particles were directed at a thin gold foil. Contrary to expectations based on Thomson's plum pudding model, some alpha particles were deflected at large angles, and a few even bounced back. Rutherford interpreted these results as evidence that the majority of an atom's mass and positive charge is concentrated in a small, dense nucleus. Scientific data from the gold foil experiment provided crucial insights into the structure of the atom. Rutherford's model also introduced the concept of the nuclear atom, where most of the atom's mass is concentrated in the nucleus, while electrons occupy the space around it. The Rutherford model marked a significant advancement in atomic theory and laid the groundwork for further research into the structure of the atom.
Bohr
The Bohr atomic model, proposed by the Danish physicist Niels Bohr in 1913, provided a revolutionary framework for understanding the behaviour of electrons within atoms. Bohr's model incorporated elements of classical mechanics and quantum theory to address the shortcomings of earlier atomic models, such as the Rutherford model. Central to Bohr's model was the concept of quantized energy levels for electrons. According to Bohr, electrons can only occupy certain discrete energy levels, or orbits, around the nucleus. When an electron transitions between energy levels, it emits or absorbs energy in the form of electromagnetic radiation, such as light.
The Bohr atomic model introduced several key formulas to describe the behaviour of electrons within atoms:
- Energy Levels Bohr proposed that the energy levels of electrons in atoms are quantized and can be calculated using the formula $$E_n = -\frac{R_H}{n^2}$$ where En is the energy of the electron in the nth energy level, RH is the Rydberg constant, and n is the principal quantum number representing the energy level
- Frequency of Radiation The frequency of radiation emitted or absorbed by an electron during a transition between energy levels can be determined using the formula $$f = \frac{E_f - E_i}{h}$$ where Ef if the final energy and Ei is the initial energy
- Wavelength of Radiation The wavelength of the emitted or absorbed radiation can be calculated using the formula $$\lambda = \frac{c}{f}$$ where c is the speed of light in vacuum
- Angular Momentum Bohr proposed that the angular momentum of an electron in a particular orbit is quantized and can be calculated using the formula $$L = n \frac{h}{2 \pi}$$ where n is the quantum number and h is the Planck's constant
Modern atom model
The modern atomic model, also known as the quantum mechanical model or the wave-mechanical model, describes the structure of atoms based on principles of quantum mechanics. This model has evolved from earlier models such as the Bohr model and the Rutherford model, offering a more accurate depiction of atomic behaviour. Key aspects of the modern atomic model include:
- Electron Cloud: In contrast to earlier models where electrons were depicted as orbiting the nucleus in fixed paths, the modern model views electrons as existing within regions of space called electron clouds or orbitals. These orbitals represent the probability of finding an electron in a particular region around the nucleus.
- Quantized Energy Levels: Electrons in atoms occupy specific energy levels, which are quantized and discrete. Each energy level corresponds to a specific orbital, and electrons can move between these levels by absorbing or emitting energy in discrete packets called photons.
- Principal Quantum Number (n): Each energy level in an atom is designated by a principal quantum number (n), which determines the energy and size of the orbital. Higher values of n correspond to orbitals farther from the nucleus and higher energy levels.
- Angular Momentum Quantum Number (l): Within each energy level, there are sublevels or subshells represented by the angular momentum quantum number (l). The value of l determines the shape of the orbital. For example, l = 0 corresponds to an s orbital (spherical shape), l = 1 corresponds to a p orbital (dumbbell shape), and so on.
- Magnetic Quantum Number (m_l): The magnetic quantum number (m_l) specifies the orientation of an orbital within space. Each subshell can have multiple orbitals with different orientations.
- Spin Quantum Number (m_s): Each electron within an orbital has a spin quantum number (m_s) that describes its intrinsic angular momentum or spin. Electrons can have one of two possible spin values: +1/2 or -1/2.
- Pauli Exclusion Principle: This principle states that no two electrons in an atom can have the same set of quantum numbers. This means that each orbital can hold a maximum of two electrons with opposite spins.
The modern atomic model provides a detailed understanding of atomic structure and behaviour, allowing scientists to predict and explain various phenomena such as chemical bonding, spectroscopy, and the behaviour of matter at the atomic and subatomic levels. It serves as a fundamental framework for much of modern chemistry and physics.
Orbitals
Orbitals are mathematical descriptions of the behaviour of electrons within atoms. They represent regions of space where one has a high probability of finding an electron. Here are the shapes of the four types of orbitals:
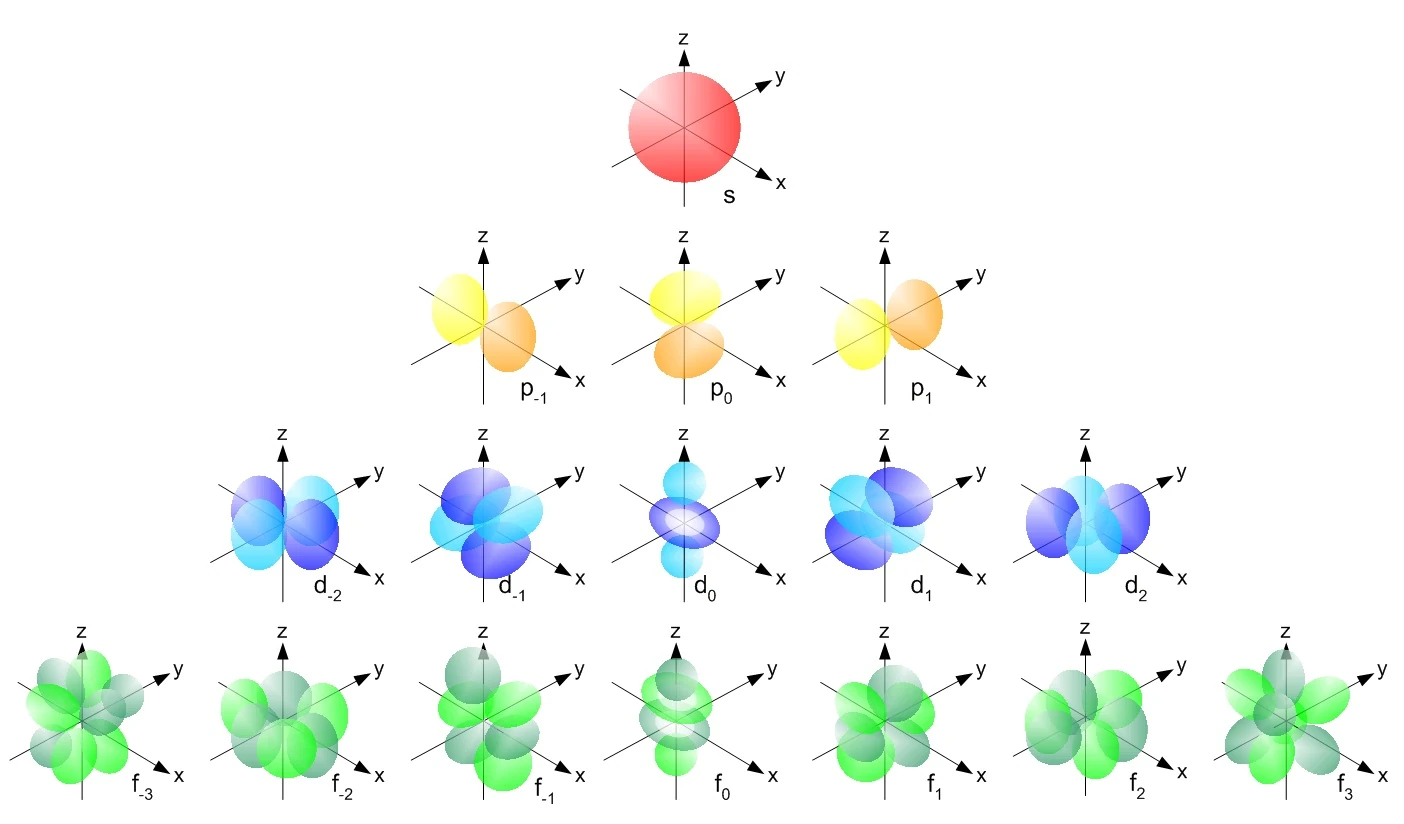
Consider that each orbital can contain two electrons only (following Pauli's exclusion principle), so you can foresee how electrons are distributed in the cloud if you know the so-called 'hybridisation' of the atom. Electrons fill the cloud following a well-defined scheme, which is shown below.
Band structure
When atoms come together to form a solid, their orbitals interact, leading to the formation of energy bands in the material's electronic structure. This is described by band theory, which is crucial for understanding the behaviour of electrons in solids. In a simplified picture, when many atoms are brought together to form a solid, the discrete energy levels of individual atoms broaden into bands due to their mutual interaction. These bands are separated by band gaps, where no electron states exist. The valence band is the highest energy band, fully occupied by electrons at absolute zero temperature, while the conduction band lies just above the valence band and is empty at absolute zero.
The electronic properties of a material are strongly influenced by its band structure. For example, conductors have partially filled or overlapping conduction and valence bands, allowing electrons to move freely and conduct electricity. Semiconductors have a small band gap between the valence and conduction bands, which can be manipulated to control their conductivity. Insulators have a large band gap, so they do not conduct electricity effectively. Band structure calculations involve complex quantum mechanical simulations and are crucial for designing new materials with desired electronic properties, such as semiconductors for electronic devices or superconductors for energy transmission. Understanding the relationship between orbitals and band structure provides insights into the behaviour of electrons in materials and drives innovation in various fields of science and technology.
Comments
Please, remember to always be polite and respectful in the comments section. In case of doubts, read this before posting.
Posted comments ⮧
Comment section still empty.
INDEX
INFO


STATISTICS

PREVIOUS ARTICLE
CONTACTS
SHARE